By Nicklas Balboa
Published in: Psychology Today
A 21st-century view of the brain.
Researchers spend decades, careers, and even lifetimes taking a closer look at how the natural world works and why certain events occur. These efforts in theory, innovation, and experimentation are the catalysts that move our society forward in the pursuit of better medicine, technology, and an understanding of the nature of humanity and life itself. As we move towards greater insight and understanding, we are reminded that progress does not always come at the speed of light, even though advances in technology make it seem that way. In our history, humans have developed forms of art, culture, medicine, architecture, government, and countless other types of rituals that reflect how much we have learned from nature.
From the discovery of the atom to the development of nuclear power, humans have gone to great lengths to understand the universe and apply this knowledge to create a better society. In light of all of this progress, there is a grey area, one final frontier that mystifies our best minds to date; the brain.
Structure Organizes and Regulates Function
The success of individual cells, which communicate and work together to form higher-order functions, depend on the quality of their surrounding organizational architecture. All things, from individual cells to the billions of people on the planet, are intimately interconnected by their respective environments.
There's more to the brain than blood vessels, cerebrospinal fluid, water, and a stem. Non-neuronal cells, such as glial cells, contribute to physical support, immune response, the blood-brain barrier, homeostasis, neuronal growth, synaptic pruning, and even regulation of neural circuits. Recent insights into the importance of two-way communication between glial cells and neurons implicate the "neurolgia" in the regulation of synapse formation and circuit connectivity1.
On the other hand, neurons are classically viewed as the principal communicators of the brain, and they are quite talkative. Neurons have specialized "conversations" through electrical and chemical activity (action potentials, neurotransmitters) via synapses, the small gaps between neurons that have the potential to facilitate or inhibit communication from the sender (presynaptic neuron) to the receiver (postsynaptic cell).
The activity of individual neurons, joined at the synapses, along with the interlacing regulatory support from neuroglia, coordinate in order to form circuits of connectivity. Neural circuits carry out functions when activated and interconnect to form larger brain networks. By better understanding the architecture of the brain’s neural circuitry, we can build a more complete "brain atlas." In order to understand the larger networks of the brain, scientists break it down into smaller components. It sounds simple enough: Utilize a biological imaging technique to study the neural activity of an organism during a specific task, then map the activity and correlate it to the task and boom — you have a map of a specific brain function.
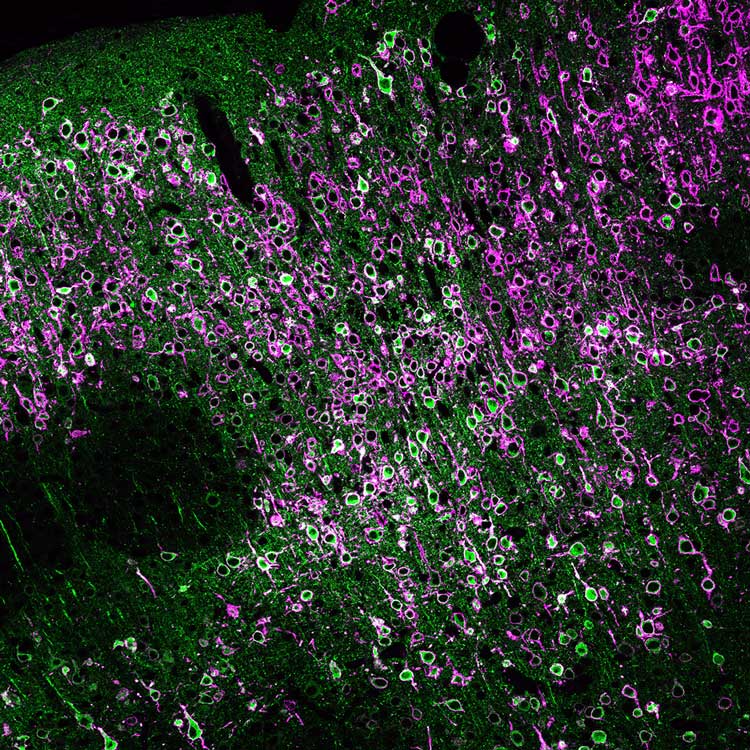
However, two defining features of the brain further compound scientist’s abilities to make accurate maps. In a recent interview with The CreatingWE Institute, Carolyn Von-Walter, Research Technician at The Bolton Lab Max Planck Florida Institute for Neuroscience, identified that both neuronal density and brain plasticity add robust layers of complexity to brain architecture.
The density of neurons creates quite the tangle of nerves in the brain. Because neurons are so tightly packed and microscopic (~300 microns), researchers are tasked with identifying which neurons are connected within a circuit, while separating the rest of the complex physiological "noise" produced in the brain.
Plasticity makes individual brains unique because of their ability to adapt to changing environments in complex, novel ways. This plasticity accounts for functional neural and hormonal responses that can be unique to their very instantiations. In other words, scientists aren’t the only ones making manipulations and keeping notes; our nervous system is flexibly adapting all of the time.
As a research technician, Carolyn is a jack-of-all-trades. She assists the lab’s principal investigator in mapping the synaptic connections in the brains of mice. The Bolton Lab, in Jupiter, Florida, aspires to understand how neural circuits are altered in neuropsychiatric disorders through state-of-the-art imaging and electrophysiological techniques. In order to study these complex connections, technicians need to be able to separate the noise from the signal, but how is this possible in such a crowded mosaic?
Viral Vectors
The brain is highly organized yet plastic, so over the course of development, brain structures exhibit components of generalized and specialized2 properties that contribute to our incredible capacities to sense, act, and think in meaningful ways.
Studying the relationship between the nervous system and behavior is a difficult task; understanding it is even more perplexing. In order to understand behavior, at is relates to neural activity, you need a reliable map of the brain.
As a neuroscientist, Carolyn’s job requires a fluency in cellular language. In order to understand where and how particular regions of interest (ROIs) operate, she utilizes viral injections to target clusters of cells within the central amygdala(e) of the mouse brain.
“These viruses are used as a tracer technology that assist in visualizing cell bodies and their axonal projections, helping to identify and define neural circuits,” says Von-Walter. After injection, the animal’s brain and subsequent neural activity are preserved in a solution of acetylcholine and cerebrospinal fluid. This ex vivo operation allows researchers to study the preserved brain for several hours, providing enough time to map the various projections that the ROI communicates with and the manner that it speaks (cell firing rate, ratio of receptors). These practices are the stepping-stones towards studying the real-time dynamics between an organism and its environment.
What Does the Future Hold?
The future of the neuroscience lies at the crossroads of technology and collaboration. As neuroscientists refine and innovate genetics and optics technologies3, they inch closer towards a live look into an organism’s brain as it operates in the natural world. By studying an animal’s neural activity in vivo, where they can freely explore their environment, researchers elucidate the dynamics of individual cells. As The Bolton Lab progresses to in vivo trials, research methods like optogenetics will decipher much richer forms of communication in the brain.
“The field of neuroscience demands transparency, communication, and feedback from the various disciplines that contribute to the scientific study of the nervous system. Part of the Max Planck initiative is to facilitate a collaborative, cutting-edge environment where scientists are supported in their research endeavors,” says Von-Walter.
Here at The CreatingWE Institute, our goal is to expand the cultural perspective of conversations. Not only do conversations provide a platform for the exchange of information, but they also hold the power to stimulate body systems and nerve pathways that influence our body’s chemistry. When this push-and-pull energy is organized and coordinated, transformational conversations emerge, where individuals come together to form incredible groups, just as the neurons in our brains form circuits.
References
1. Look who is weaving the neural web: glial control of synapse formation. Bolton, M.M., and Eroglu, C. (2009). Look who is weaving the neural web: glial control of synapse formation. Curr. Opin. Neurobiol. 19, 491–497.
2. Regional Specialization of Pyramidal Neuron Morphology and Physiology in the Tree Shrew Neocortex Parra, A., Baker, C.A., and Bolton, M.M. (2019). Regional Specialization of Pyramidal Neuron Morphology and Physiology in the Tree Shrew Neocortex. Cerebral Cortex, 1-18.
3. Cellular resolution circuit mapping in mouse brain with temporal-focused excitation of soma-targeted channelrhodopsin. Christopher A. Baker, Yishai M. Elyada, Andres Parra-Martin, McLean Bolton. eLIFE, August 15, 2016 DOI: http://dx.doi.org/10.7554/eLife.14193